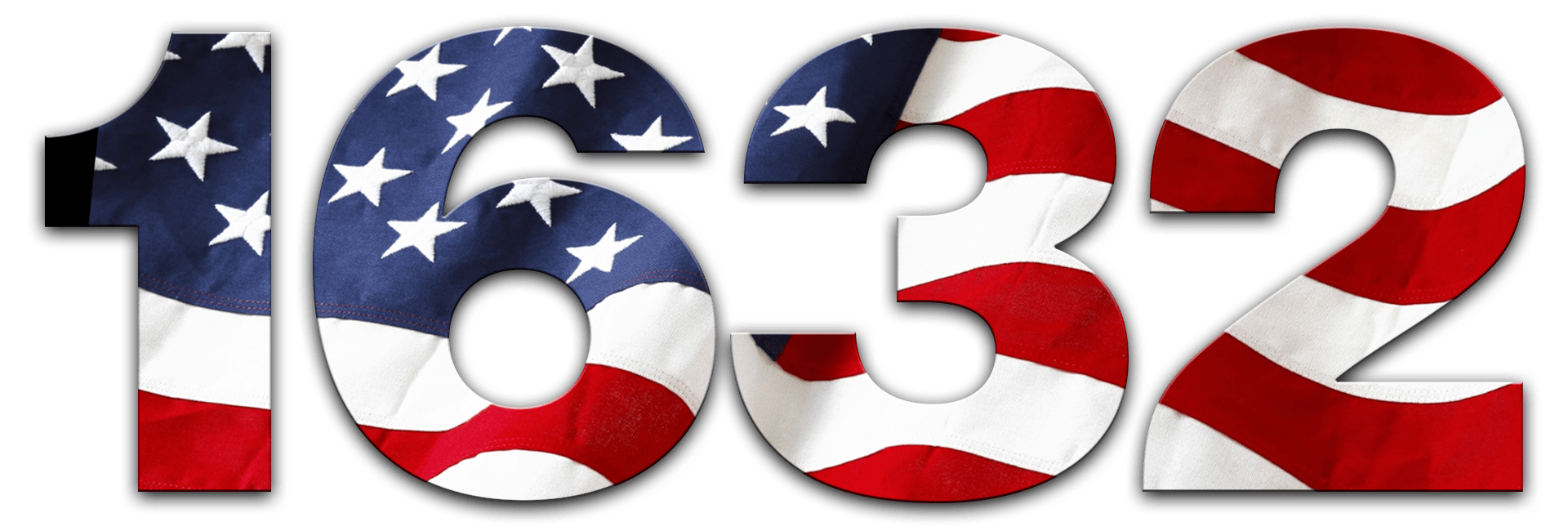
Welcome!
This website is the official hub for all information related to Eric Flint’s 1632 series (aka Ring of Fire series), including information for prospective authors. Assembled here are all the technical information, archives, and files of interest to the happy habitants of the Baen’s Bar 1632 Group. You are invited to browse this site, get involved in the discussion groups and even download a free e-book version of the novel that started it all – 1632.
Feel free to browse around the site for files and pages of interest to Barflies in general and those who would write in the 1632 Universe. If you want to include an up-timer in your story, you need to get your character from Virginia’s Grid.
Eric says you can make up down-timers because there’s milyuns and milyuns of them. But famous down-timers … he may have plans for.
Potential authors should first read Iver P. Cooper’s essay “So You Want to Write a Grantville Gazette Story?,” and the rest of the Author’s Manual files. You should also peruse Rick Boatright’s “Dead Horses” article to see if your idea has been flogged to a pile of dog food.
In any case, You are welcome to browse, lurk, participate, smoke, maybe burst into glorious song or even flames. Stranger things have happened here … recently!
Use the search bar or click one of the menu links to begin.